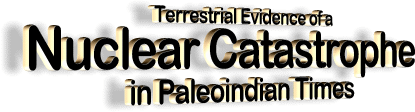
by Richard B. Firestone &
William Topping
Mammoth Trumpet
Volume 16, Number 2 March 2001
from
CenterFirstAmericans Website
The Paleoindian occupation of North
America, theoretically the point of entry of the first people to the
Americas, is traditionally assumed to have occurred within a short
time span beginning at about 12,000 yr B.P. This is inconsistent
with much older South American dates of around 32,000 yr B.P.1
and the similarity of the Paleoindian toolkit to Mousterian
traditions that disappeared about 30,000 years ago.2
A pattern of unusually young radiocarbon dates in the Northeast has
been noted by Bonnichsen and Will.3, 4
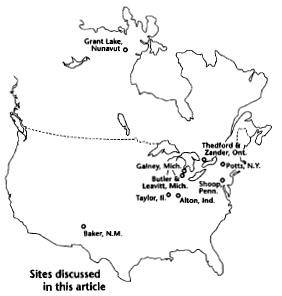
Our research indicates that the entire Great Lakes region (and
beyond) was subjected to particle bombardment and a catastrophic
nuclear irradiation that produced secondary thermal neutrons from
cosmic ray interactions. The neutrons produced unusually large
quantities of 239Pu and substantially altered the natural
uranium abundance ratios (235U/238U) in
artifacts and in other exposed materials including cherts,
sediments, and the entire landscape. These neutrons necessarily
transmuted residual nitrogen (14N) in the dated charcoals
to radiocarbon, thus explaining anomalous dates.
The evidence
from dated materials
We investigated a cluster of especially young radiocarbon dates
concentrated in the north-central area of North America. For
example, at the Gainey site in Michigan a 2880 yr B.P. radiocarbon
date was reported, while the thermoluminescence date for that site
is 12,400 yr B.P.5
Other anomalous dates found at Leavitt in Michigan,6
Zander and Thedford in Ontario,7
Potts in New York,8
Alton in Indiana,9
and Grant Lake in Nunavut10
are summarized in Table 1.
The Grant Lake Paleoindian site is most
remarkable because its 160 [rc] yr B.P. age is nearly contemporary,
while adjacent and deeper samples give ages of 1480-3620 [rc] yr B.P.
Stratigraphic associations place Paleoindian occupations at depth on
the prehistoric North American landscape on sediments that form the
old C horizon composed of parent material, Wisconsinan deposits that
predate Holocene sediment buildup.11,12,13
The young Paleoindian dates cannot be correct, particularly since
there are no patterned anomalies noted in later-period prehistoric
assemblages relating to higher stratigraphic positions.
In a pioneering study of the Paleoindian
site at Barnes, Michigan, Wright and Roosa observed that Paleoindian
artifacts were deposited before the formation of spodosols ceased in
this area about 10,000 yr B.P.14
This conclusion was based on observing that cemented
sediments on artifacts, found outside their original context,
defines their original stratigraphic position.
The evidence
from particle bombardment
Sediment profiles were taken at Paleoindian sites and at numerous
widely separated control locations in Michigan. The C sediment
horizon is clearly recognized by its transitional color and
confirmed by elevated concentrations of potassium and other
isotopes. Color and chemistry are key indicators of this very old
soil11,12,13,14
derived from parent materials and associated postglacial runoff.15
At Gainey, large quantities of micrometeorite-like
particles appear to be concentrated near the boundary between the B
and C sediment horizons.
They can be separated with a magnet and
are identified by the presence of chondrules and by visual evidence
of sintering and partial melting. These particles, dissimilar to
common magnetites, are found in association with a high frequency of
"spherules." The depth profiles for potassium and particles at the
Gainey site are compared in Fig. 1. Minor vertical sorting of
particles is apparent, with a shallow spike of particles near the
surface probably resulting from modern agricultural or industrial
activity. Total gamma-ray counting of sediment profiles in the
various locations invariably showed increased radioactivity at the
B-C boundary consistent with enhanced potassium (40K) and
possibly other activities.
Microscopic examination of chert artifacts from several widely
separated Paleoindian locations in North America revealed a high
density of entrance wounds and particles at depths that are evidence
of high-velocity particle bombardment. Chondrules were identified
visually; their presence necessarily indicates heating during
high-speed entry into the atmosphere.
The depth of penetration into the
artifacts implies that the particles entered with substantial
energy.16 Field
simulations with control cherts for large particles (100-200
microns) suggest an entrance velocity greater than 0.4 km/s, and
experiments at the National Superconducting Cyclotron Laboratory
indicate that the smaller particles left tracks comparable to about
526 MeV iron ions (56Fe) in Gainey artifacts. Similar
features are not observed in later-period prehistoric artifacts or
in bedrock chert sources. Track angles were estimated visually;
track densities were measured with a stage micrometer; track depths
were found by adjusting the microscope focus through the track.
These data are summarized in Table 1.
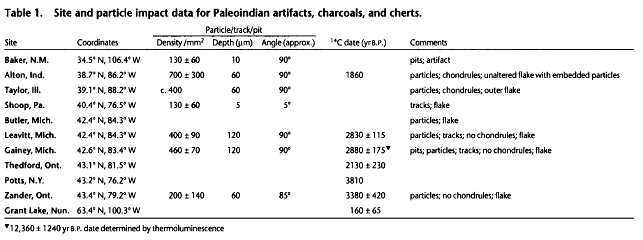
Track and particle data in Table 1 suggest that the total track
volume (density times depth) is highest at the Michigan, Illinois,
and Indiana sites and decreases in all directions from this region,
consistent with a widespread catastrophe concentrated over the Great
Lakes region. The nearly vertical direction of the tracks left by
particle impacts at most sites suggests they came from a distant
source.
A barn is a unit of area equal to 10-24 cm2,
used in nuclear physics. The fraction of isotopes that are
transformed by a nuclear reaction is given by s x I, where s is the
cross section in cm2 of the target presented by an atom,
and I is the neutron flux per cm2 impinging on the
target. Most neutron-induced reactions involve the capture of a
neutron to produce a heavier isotope of the same element. Exceptions
include 14N, which captures a neutron and emits a proton
to produce 14C; and 235U, which mainly
fissions into two lighter elements. The relative size of isotopes in
chert is shown in figure "A neutron's view of chert."
The evidence
from uranium and plutonium
Natural uranium, which is ubiquitous in cherts, has a 235U/238U
isotopic ratio of 0.72 percent, which varies by less than 0.1
percent in natural sources.17
Significant variations in the isotopic ratio do not occur because of
chemical processes; however, a thermal neutron bombardment depletes
235U and thus alters the ratio. Solar or galactic cosmic rays
interacting with matter produce fast secondary neutrons that become
thermalized by scattering from surrounding materials.
Thermal neutrons see a target of large
cross section (681 barns) A for destroying 235U, compared with a
target of only 2.68 barns for neutron capture on 238U. Therefore,
despite the low abundance of 235U, about 1.8 times as many 235U
atoms are destroyed as 238U atoms by thermal neutrons.
If a large cosmic-ray bombardment impacted the earth and irradiated
the prehistoric landscape with thermal neutrons, the 235U/238U ratio
would be changed; 239Pu would be produced from neutron
capture on 238U, followed by the decay of 239U. Neutrons colliding
with nitrogen (1.83 barns) would create 14C in exactly the same way
14C is normally produced in the upper atmosphere, necessarily
resetting the radiocarbon dates of any organic materials lying near
the surface on the North American prehistoric landscape--including
charcoals at Paleoindian sites--to younger values.
239Pu produced during the
bombardment will also be partly destroyed by thermal neutrons with
1017 barn cross section. Assuming 239Pu
doesn't mobilize, it will decay back to 235U (half-life 24,110 yr),
partially restoring the normal abundance.
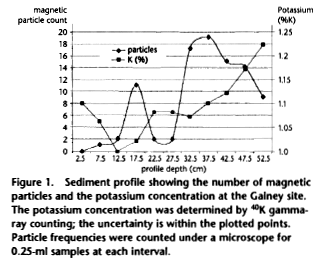
Paleoindian artifacts from Gainey, Leavitt, and Butler, and two
later-period artifacts from the same geographic area of Michigan
were analyzed for 235U content by gamma-ray counting at the
Phoenix
Memorial Laboratory, University of Michigan. They were compared with
identical chert types representative of the source materials for the
artifacts. Control samples were extracted from the inner core of the
purest chert known to be utilized by prehistoric people.
The Paleoindian artifacts contained
about 78 percent as much 235U as the controls and later-period
artifacts, suggesting substantial depletion. Depletion of 235U
necessarily indicates that thermal neutrons impacted these artifacts
and the surrounding prehistoric landscape.
Various artifacts, cherts, sediments, and a control sample
containing about 0.2 percent uranium obtained from uraninite were
sent to the McMaster University Centre for Neutron Activation
Analysis to determine 235U concentration by delayed neutron counting
and 238U concentration by activation analysis. These results are
shown in Table 2. The 235U/238U ratios for all samples except the
control deviated substantially from the expected ratio. McMaster ran
additional calibration standards and has considerable expertise
analyzing low-level uranium.
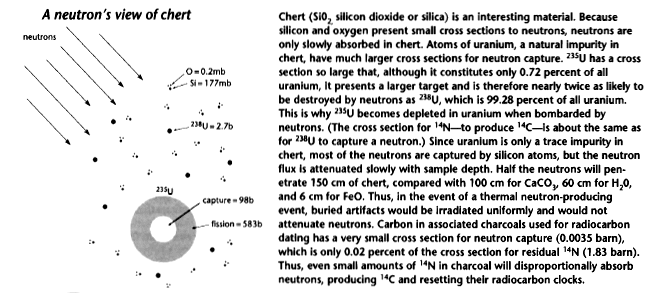
This analysis was sensitive to a few ppb
for 235U and 0.1-0.3 ppm for 238U, more than sufficient to precisely
analyze the uranium-rich chert samples (0.7-163.5 ppm). Most samples
were depleted in 235U, depletion increasing geographically from the
southwest (Baker, Chuska chert, 17 percent) to the northeast (Upper
Mercer, 77 percent), as shown in Table 2.
This is consistent with
cosmic rays focused towards northern latitudes by Earth's magnetic
field. Only a very large thermal neutron flux, greater than 1020
n/cm2, could have depleted 235U at all locations.
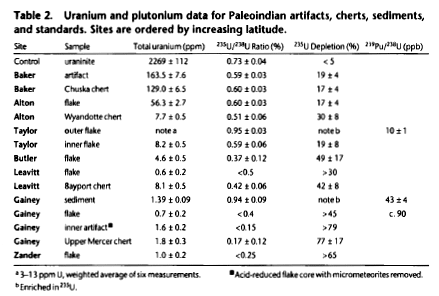
Samples of unaltered flakes from Taylor and sediment originally
adjacent to Gainey artifacts showed 235U enriched by 30 percent.
Both samples were closely associated with the particles described
above. The position of these samples appears to be related to the
enrichment, which cannot be explained by thermal neutrons from the
bombardment. To test this, we bathed another Taylor flake in
48-percent HF at 60°F for ten minutes to remove the outer 70 percent
of the sample and the attached particles. Analysis showed the
"inner" flake depleted in 235U by 20 percent, consistent with the
other depleted cherts.
Samples of Gainey sediment and Taylor flakes were analyzed for
plutonium by Nuclear Technology Services, Inc., of Roswell, Georgia,
which specializes in radiochemistry using standard methodology. The
plutonium, with an aliquot of NIST-traceable 242Pu added, was
chemically separated on an anion exchange resin column and counted
on an alpha-particle spectrometer. The 239Pu/238U
ratios in both samples were approximately 10 ppb, vastly exceeding
the expected ratio of 0.003 ppb.18
The results of this analysis are shown in Table 2.
Chert is a glass-like material highly impervious to penetration by
any nuclear fallout that might also contribute 239Pu. We
analyzed a long-exposed piece of Bayport chert by gamma-ray counting
at the LBNL low-background facility for the presence of cesium-137
(137Cs), a key indicator of fallout (from nuclear testing), and
found none. The B-C interface typically lies sufficiently deep that
contamination by fallout is improbable. It is important to note that
fallout cannot explain the depletion of 235U.
Since the depletion of 235U must have resulted from bombardment by
thermal neutrons, the presence of 239Pu from irradiation
of 238U is expected. The total thermal neutron flux required to
produce the observed 239Pu concentration can be calculated from the
relative concentrations of 239Pu (corrected for the
decay) and 238U, and the thermal neutron-capture cross section for
238U. This neutron flux can then be used to estimate the amount of
additional 14C that would have been produced in charcoal by neutrons
colliding with 14N (14N cross section = 1.83 barns).
The corrected radiocarbon age can then
be estimated by comparing the current amount of 14C in the dated
charcoals, determined from their measured radiocarbon age, with the
amount of 14C that would have been produced by the bombardment. For
these calculations we assume that charcoal contains 0.05 percent
residual nitrogen19
and that initial 14C concentrations were the same as today (one 14C
atom for 1012 12C atoms).
We derive a thermal neutron flux of c. 1017 n/cm2 at Gainey, which
corresponds to an approximate date of 39,000 yr B.P. No radiocarbon
date is available for the more southerly Taylor site, but for the
conventional range of accepted Paleoindian dates the neutron flux
would be c. 1016 n/cm2, giving a date of about
40,000 yr B.P. These calculations necessarily neglect differences in
the neutron flux experienced by the dated charcoal and the
artifacts, the effects of residual 239Pu from previous bombardments,
and loss of 239Pu due to leaching from chert over time.
The neutron flux calculated from the 235U/238U ratio is more than
1000 times that implied by the level of 239Pu. Since 239Pu decays to
235U, partly restoring the natural abundance, it appears that
substantial quantities of 239Pu have migrated out of the chert. This
mobility is demonstrated at the Nevada Test Site, where plutonium,
produced in nuclear tests conducted by the U.S. between 1956 and
1992, migrated 1.3 km.20
It has also been shown that atoms
produced by radioactive decay or nuclear reaction become weakly
bound to the parent material and pass more readily into solution
than isotopes not affected.21
Both 239Pu and 235U are thus expected to be mobile,
complicating any analysis. This is consistent with the enrichment of
235U in the two external samples where migrating 239Pu or
235U may have been trapped, thus enriching the relatively
uranium-poor outer regions. Alternatively, excess 235U may have been
carried in by the particles. Radiocarbon produced in situ by
irradiation should also be mobile. If 14C is more mobile than
239Pu, then the dates calculated above should be decreased
accordingly.
Redating North
American sites
The 39,000 yr B.P. date proposed for the Gainey site is consistent
with the prevailing opinion among many archaeologists about when the
Americas were populated. It is also commensurate with dates for
South American sites and with a Mousterian toolkit tradition that
many see as the Paleoindian precursor.
The proposed date for the Gainey site
also falls closer in line with the radiocarbon date for a
Lewisville, Texas, Paleoindian site of 26,610 ± 300 yr B.P.22,23
and radiocarbon dates as early as c. 20,000 yr B.P. for Meadowcroft
Rockshelter.24 Since
the Lewisville and Meadowcroft sites were likely exposed at the same
time to thermal neutrons, we estimate that their dates should be
reset to c. 55,000 yr B.P. and c. 45,000 yr B.P., respectively.
It is likely that Paleoindians occupied low latitudes during the
full glacial and migrated to more northerly areas as the ice front
retreated. Therefore the pattern of dates makes sense from the
archaeologist's point of view. Dates for North American sites should
generally be reset by up to 40,000 years, depending on latitude and
overburden.
Geologists believe that before c. 15,000 yr B.P. the Wisconsinan
glaciation covered the more northerly locations where Paleoindian
sites have been found.25
The ice sheet would have shielded the landscape and any artifacts
from an irradiation.
(The Gainey thermoluminescence date of 12,400
yr B.P. is probably a result of the heat generated by the nuclear
bombardment at that time, which would have reset the TL index to
zero.)
The modified dates for Paleoindian settlements suggest that
the timetable for glacial advance sequences, strongly driven by
conventional radiocarbon dates, should be revisited in light of the
evidence presented here of much older occupations than previously
thought."
The alignment of magnetic particles in sediment indicates that the
Earth's magnetic poles have repeatedly reversed their polarity in
the past. Complete magnetic excursions occurred about 10 times in
4.5 million years; the last reversal occurred about 700,000 years
ago. Magnetic excursions occur every 10,000-20,000 years when the
Earth's magnetic field becomes weak, and the poles may even reverse
for a short time.
The evidence
from tree rings and marine sediments
A large nuclear bombardment should have left evidence elsewhere in
the radiocarbon record. It is well known that radiocarbon dates are
increasingly too young as we go back in time. The global Carbon
Cycle suggests that 14C produced by cosmic rays would be rapidly
dispersed in the large carbon reservoirs in the atmosphere, land,
and oceans.26
We would expect to see a sudden increase
in radiocarbon in the atmosphere that would be incorporated into
plants and animals soon after the irradiation; after only a few
years, most of the radiocarbon would move into the ocean reservoirs.
The 14C level in the fossil record would reset to a higher value.
The excess global radiocarbon would then decay with a half-life of
5730 years, which should be seen in the radiocarbon analysis of
varved systems.
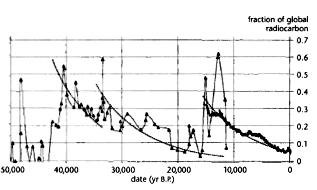
Fig. 2 plots 14C from the INTCAL98 radiocarbon age calibration data
of Stuiver et al. for 15,000-0 yr B.P.27
and Icelandic marine sediment 14C data measured by Voelker et al.
for 50,000-11,000 yr B.P.28
Excess 14C is indicated by the difference between the reported
radiocarbon dates and actual dates. Sharp increases in 14C are
apparent in the marine data at 40,000-43,000, 32,000-34,000 and c.
12,000 yr B.P
These increases are coincident with
geomagnetic excursions B that occurred at about 12,000 (Gothenburg),
32,000 (Mono Lake), and 43,000 yr B.P. (Laschamp),29
when the reduced magnetic field would have made Earth especially
vulnerable to cosmic ray bombardment. The interstitial radiocarbon
data following the three excursions were numerically fit, assuming
exponential decay plus a constant cosmic ray-produced component. The
fitted half-lives of 5750 yr (37,000-34,000 yr B.P.), 6020 yr
(32,000-16,000 yr B.P.), and 6120 yr (12,000-0 yr B.P.) are in good
agreement with the expected value.
We also determined that contemporary radiocarbon contains about 7
percent residual 14C left over from the catastrophe. The constant
cosmic ray production rate was about 34 percent higher for the
Icelandic sediment than the INTCAL98 samples, perhaps implying
higher cosmic ray rates farther north. Disregarding fluctuations in
the data from variations in ocean temperatures and currents, the
results are clearly consistent with the decay of radiocarbon
following the three geomagnetic excursions.
In Fig. 2, the sharp drop in 14C activity before 41,000 yr B.P.
suggests that global radiocarbon increased by about 45 percent at
that time and by about 20 percent at 33,000 and 12,000 yr B.P The
results are remarkably consistent with Vogel's comparison of 14C and
U-Th dates of a stalagmite that indicates global radiocarbon
increased about 75 percent from 30,000 to 40,000 yr B.P. and about
30 percent around 18,000 yr B.P.30
McHargue et al. found high levels of 10Be in Gulf of
California marine sediments at 32,000 and 43,000 yr B.P.C that could
not be explained by magnetic reversal alone and were attributed to
cosmic rays, possibly from a supernova.29 The geomagnetic excursion
at 12,500 yr B.P. coincides with the thermoluminescence date from
Gainey, and additional evidence for a cosmic ray bombardment at that
time is found in the increases of 10Be,31
Ca,32 and Mg32
in Greenland ice cores around 12,500 yr B.P. Similar increases are
also seen in the data for NO3-, SO4-, Mg+, Cl-, K+, and Na+ ions in
Greenland ice cores.33
This occurrence can be dated precisely
to 12,500 ± 500 yr B.P., an average of the remarkably consistent
concentration peak centroids in the Greenland ice core data.
Significant increases at that time are not found in comparable data
for the Antarctic, which indicates that the cosmic ray irradiation
was centered in the Northern Hemisphere. Weak evidence of an
occurrence at 12,500 yr B.P. is seen in the radiocarbon record for
marine sediments near Venezuela,34
confirming that the cosmic ray bombardment was most severe in
northern latitudes.
Lunar cosmogenic data also show evidence of increased solar cosmic
ray activity at or before 20,000 yr B.P.35,36
although these data are not sensitive to earlier irradiation.
Beryllium occurs naturally as 9Be. 10Be is
produced by cosmic rays, mostly protons, striking the atmosphere and
breaking apart nitrogen and oxygen. It has a half-life of 1.5
million years. Unlike 14C, which is caught up in the global Carbon
Cycle, 10Be is inert and falls as dust. 10Be
is produced almost entirely by galactic cosmic rays, which are much
higher in energy than solar cosmic rays.
Thus any increase in 10Be
would be cosmic in origin; and the cosmic ray rate could only change
if there were a nearby supernova. During the last Ice Age the
10Be deposition rate in ice at both poles was much higher than
today. Gulf of California marine sediments clearly show strong
10Be peaks at 32,000 and 43,000 yr B.P. McHargue argues
that these peaks can only be explained by a supernova.
The effect of a
supernova on Earth
Sonett suggests that a single supernova would produce two or three
shock waves, an initial forward shock and a pair of reverse shocks
from the initial expansion and a reflected wave from the shell
boundary of a more ancient supernova.39,40
Fig. 2 shows that each episode in a series produced a similar amount
of atmospheric radiocarbon.
The sun lies almost exactly in the
center41 of the Local
Bubble, believed to be the result of a past nearby supernova event.
A candidate for the reverse shock wave is the supernova remnant
North Polar Spur, with an estimated age of 75,000 years and a
distance of 130 ± 75 parsecs (424 light years),42
conveniently located in the north sky from where it would have
preferentially irradiated the Northern Hemisphere.
Assuming the Taylor flux is average and
1,000 neutrons are produced per erg of gamma-ray energy,43
the catastrophe would have released about 1016 erg/cm2
(2 x 108 cal/cm2), corresponding to a solar
flare of 1043 ergs or a gamma-flash of 1054
ergs from a supernova about 1 parsec away.
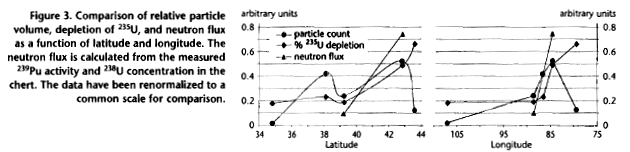
The geographical distribution of particle tracks, 235U
depletion, and 239Pu concentration shown in Fig. 3 are
quite consistent, although the particle tracks seem to be confined
to a smaller geographic area. They indicate energy released over the
northeastern sector of the U.S., with maximum energy at about 43° N,
85° W, the Michigan area of the Great Lakes region.
A history of
suspected cosmic cataclysms over the ages
Wdowczyk and Wolfendale 44
and Zook 36 propose,
based on the existing record of solar flare intensities, that solar
flares as large as 3 x 1038 ergs should be expected every
100,000 years. Clark et al. estimate that supernovas release 1047-1050
ergs within 10 parsecs of Earth every 100 million years.45
Brackenridge suggests that a supernova impacted the earth in
Paleoindian times.46
Damon et al. report evidence from the
14C tree ring record that SN1006, which occurred at a distance of
1300 parsecs, produced a neutron shower of 2 x 108 n/cm2.47
Castagnoli et al. report evidence of the past six nearby supernovae
from the thermoluminescence record of Tyrrhenian sea sediments.48
Dar et al. suggest that a cosmic ray jet
within 1000 parsec would produce 1012 muons/cm2 (greater than 3 x
109 eV) and 1010 protons and neutrons/cm 2 (greater than 106 eV) and
deposit over 1012 erg/cm2 in the atmosphere every 100 million years.49
A cosmic ray jet is also predicted to produce heavy elements via the
r-process and could be a source of 235U enriched up to 60 percent in
uranium.
The Paleoindian catastrophe was large by standards of all suspected
cosmic occurrences. Normal geomagnetic conditions would focus cosmic
rays towards the magnetic poles, concentrating their severity in
those regions. However, low magnetic field intensity during a
geomagnetic excursion may have allowed excessive cosmic rays to
strike northeastern North America. (Whether the geomagnetic
excursion admitted cosmic radiation, or the radiation caused the
excursion, is uncertain. Given our present state of knowledge, cause
and effect in this instance are unclear.)
The presence of a nearby small and dense
interstellar cloud may explain the origin of the particle
bombardment.50 The
size of the initial catastrophe may be too large for a solar flare,
but a sufficiently powerful nearby supernova or cosmic ray jet could
account for it. It appears that the catastrophe initiated a sequence
of events that may have included solar flares, impacts, and
secondary cosmic ray bombardments.
A devastating
effect on Earth
The enormous energy released by the catastrophe at 12,500 yr B.P.
could have heated the atmosphere to over 1000°C over Michigan, and
the neutron flux at more northern locations would have melted
considerable glacial ice. Radiation effects on plants and animals
exposed to the cosmic rays would have been lethal, comparable to
being irradiated in a 5-megawatt reactor more than 100 seconds.
The overall pattern of the catastrophe matches the pattern of mass
extinction before Holocene times. The Western Hemisphere was more
affected than the Eastern, North America more than South America,
and eastern North America more than western North America.51,52,53
Extinction in the Great Lakes area was more rapid and pronounced
than elsewhere.
Larger animals were more affected than
smaller ones, a pattern that conforms to the expectation that
radiation exposure affects large bodies more than smaller ones.54,55
Sharp fluctuations of 14C in the Icelandic marine sediments at each
geomagnetic excursion are interesting; because global carbon
deposits in the ocean sediments at a rate of only about 0.0005
percent a year, a sudden increase in sediment 14C may reflect the
rapid die-off of organisms that incorporated radiocarbon shortly
after bombardment.
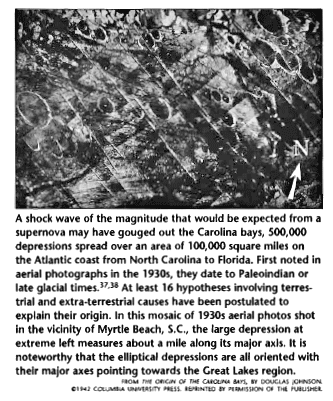
Massive radiation would be expected to cause major mutations in
plant life. Maize probably evolved by macro-mutation at that time,55,56
and plant domestication of possibly mutated forms appears worldwide
after the Late Glacial period. For example, there was a rapid
transition from wild to domesticated grains in the Near East after
the catastrophe.57
Implications
for future study
Much of what we assume about the Paleoindian period and the peopling
of the Americas has been inferred from conventional radiocarbon
chronology, which often conflicts with archaeological evidence. This
work mandates that conventional radiocarbon dates be reinterpreted
in light of hard terrestrial evidence of exposure of the radiocarbon
samples to a cosmological catastrophe that affected vast areas of
North America and beyond.
A nuclear catastrophe can reset a group
of unrelated artifacts to a common younger date, creating gaps and
false episodes in the fossil record. Geographical variation and
complicated overburdens may further confuse the interpretation.
Scrutiny of Paleoindian artifacts and the North American paleolandscape, associated stratigraphic sediments, coupled with
continued radiological investigations, may provide more evidence for
the cosmic catastrophe and new clues to the origin of Paleoindians.
References
1 Gruhn, R., in Clovis: Origins and
Adaptations, R. Bonnichsen, K. L. Turnmire, eds. (Oregon State
University Press, Corvallis, 1991), pp. 283-286.
2 Muller-Beck, H., Science 152, 1191 (1985).
3 Bonnichsen, R., in Clovis: Origins and Adaptations, R.
Bonnichsen, K. L. Turnmire, eds. (Oregon State University Press,
Corvallis , 1991), pp. 309-329.
4 Bonnichsen, R., F. Will, in Ice Age Peoples of North America,
R. Bonnichsen, K. L. Turnmire, eds. (Oregon State University
Press, Corvallis, 1999), pp. 395-415.
5 Simons, D. B., M. J. Shott, H. T. Wright, Arch. East. Nor.
Amer. 12, 266 (1984).
6 Shott, M.J., The Leavitt Site (Museum of Anthropology, Ann
Arbor, 1993).
7 Stewart, A., Ontario Arch. 41, 45 (1984).
8 Gramly, R. M., J. Lothrop, Arch. East. Nor. Amer. 12, 1222
(1984).
9 Tomak, C. H., Dancey Ohio Arch. Coun., Columbus, 117 (1994).
10 Wright, J. V., Borden Number Kkln-2, Lab No. S-833. Canadian
Archaeology Association C14 Database Search,
http://www.canadianarchaeology.com/localc14/c14search.htm
11 S. Boggs, S., Principles of Sedimentology and Stratigraphy (MacMillan,
New York, 1987).
12 Easterbrook, D. J., Surface Processes and Landforms (MacMillan,
New York, 1993).
13 Birkeland, P. W., Soils and Geomorphology (Oxford University
Press, New York, 1984).
14 Wright, H. T., W. B. Roosa, American Antiquity 31, 850
(1966).
15 Turner, M. D., E. J. Zeller, G. A. Dreschoff, J. C. Turner,
in Ice Age Peoples of North America, R. Bonnichsen, K. L.
Turnmire, eds. (Oregon State University Press, Corvallis, 1999),
pp. 42-77.
16 firestone, R. B., W. Topping, Paleoindian Nuclear Event,
http://ie.lbl.gov/Paleo/paleo.html
17 Kuroda, P. K., The Origin of the Chemical Elements,
(Springer-Verlag, Berlin Heidelberg, 1982).
18 Seaborg, G. T., W. D. Loveland, The Elements beyond Uranium,
(John Wiley & Sons, Inc., New York, 1990).
19 Ostrom, N., analysis at Michigan State University of charcoal
and wood dated at 2800 and 42,000 yr B.P. respectively, private
communication.
20 Kersting, A. B., et al., Nature 397, 56 (1999).
21 Cherdyntsev, V. V., Abundance of Chemical Elements. (The
University of Chicago Press, Chicago, translated by W.
Nichiporuk, 1961).
22 Wormington, H. M., Ancient Man in North America, (The Denver
Museum of Natural History, Denver, 1957).
23 Shirley, R. H., et al., Environmental Geology Notes 109,
1985.
24 Adovasio, J. M., R. C. Carlisle, Science 239, 713 (1988).
25 Farrand, W. R., The Glacial Lakes around Michigan, Bulletin
4. (Geological Survey Division, Michigan Department of
Environmental Quality, 1988).
http://www.deq.state.mi.us/gsd/Gltext.html
26 Schimel, D. S., et al., in Climate Change 1994. Radiative
Forcing of Climate Change and An Evaluation of the IPCC IS92
Emission Scenarios, J. T. Houghton, L. G. M. Filho, J. Bruce, H.
Lee, B. A. Callander, E. Haites, N. Harris, and K. Maskell, eds.
(IPCC Report. Cambridge University Press, Cambridge, 1994).
27 Stuiver, M., et al., Radiocarbon 40, 1041 (1998).
28 Voelker, A. H. L., et al., Radiocarbon 40, 517 (1998).
29 McHargue, L. R., P. E. Damon, D. J. Donahue, Geophys. Res.
Lett. 22, 659 (1995).
30 Vogel, J. C., Radiocarbon 25, 213 (1983).
31 Finkel, R. C., K. Nishiizumi, J. Geophys. Res. 102, 26699
(1997).
32 De Angelis, M., J. P. Steffensen, M. R. Legrand, H. B.
Clausen, C. U. Hammer, Journal of Geophysical Research 102,
26681 (1997).
33 Mayewski, P. A., et al., Journal of Geophysical Research 102,
26345 (1997).
34 Hughen, K. A., et al., Radiocarbon 39, 483 (1998).
35 Jull, A. T., et al., Geochimica et Cosmochimica Acta 62, 3025
(1998).
36 Zook, H. A., Proc. Conf. Ancient Sun, J. A. Eddy, R. Merrill,
eds., 245 (1980).
37 Prouty, W. F., Geol. Soc. Am. Bull. 63, 167 (1952).
38 Eyton, J. R., J. L. Parkhurst, A Re-Evaluation of the
Extraterrestrial Origin of the Carolina Bays,
http://abob.libs.uga.edu/bobk/cbayint.html
(1975).
39 Sonett, C. P., G. E. Morfill, J. R. Jokipii, Nature 330, 458
(1987).
40 Sonett, C. P., Radiocarbon 34, 239 (1992).
41 Davelaar, J., J. A. M. Bleeker, A. J. M. Deerenberg, Astron.
Astrophys. 92, 231 (1980).
42 Lingenfelter, R. E., R. Ramaty, in Radiocarbon Variations and
Absolute Chronology, I. U. Olson, ed. (John Wiley & Sons, New
York, 1970), pp. 513-537.
43 Wdowczyk, J., A.W. Wolfendale, Nature 268, 510 (1977).
44 Clark, D. H., W. H. McCrea, F. R. Stephenson, Nature 265, 318
(1977).
45 Brackenridge, G. R., Icarus 46, 81 (1981).
46 Damon, P. E., D., Kaimei, G. E. Kocharov, J. B. Mikheeva, A.
N. Peristykh, Radiocarbon 37, 599 (1995).
47 Castagnoli, G. C., G. Bonino, and S. Miono, Nuovo Cimento 5C,
488 (1982).
48 Dar, A., A. Laor, N. J. Shaviv, Phys. Rev. Lett. 80, 5813
(1998).
49 Frisch, P. C., American Scientist 88 (2000).
50 Guilday, J. E., P. S. Martin, Pleistocene Extinctions, the
Search for a Cause, P. S. Martin, H. E. Wright, eds. (Yale
University Press, New Haven,1967) pp. 5-120.
51 Meltzer, D. J., T. I. Mead, Quat. Res. 19, 130 (1983).
52 Robinson, A., Earth Shock (Thames and Hudson, Ltd, London,
1993).
53 Farrand, W. R., Science 133, 729 (1961).
54 Sanderson, I. T., Sat. Evening Post 232, 82 (1960).
55 Iltis, H. H., Science 222, 886 (1983).
56 Benz, F. F., H.H. Iltis, Amer. Antiq. 55, 500 (1990).
57 Murray, J., The First European Agriculture (Edinburgh
University Press, Edinburgh, 1970).
|